Flash and JavaScript are required for this feature.
Download the video from Internet Archive.
Description
This lecture introduced different aircraft systems.
Instructor: Philip Greenspun, Tina Srisvastava
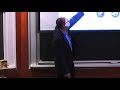
Lecture 4: Aircraft Systems
PHILIP GREENSPUN: All right, so a complicated aircraft will have a lot of systems. These are the systems from the Canadair Regional Jet. How well did that I fly it? We landed it once in Toronto, and I was doing this, and the captain said to me, well-- he was a young guy-- he said, well, you know nobody was born knowing how to fly a 53,000-pound jet. But anyway, this is the kind of stuff you have to learn for the big aircraft.
Here are these systems that we're going to talk about that you have on a small aircraft. The engine, first of all, what are the types? You might remember the cool old days. You had these big radio engines, and they went up to 3,500 horsepower, I think, was the biggest one. But your typical World War Ii fighter might have a 2000 horsepower-ish radial engine. Turboprops, so we'll get into this more. I have a special lecture on turbines and multi-engine.
But it's a jet engine that's hooked into a propeller, and that lets you land on shorter runways, and it's more fuel-efficient at lower altitudes. The popular examples, the Pratt & Whitney PT6 has been around since the 50s. A competitor from the similar era was designed by Garrett. Those have typically slugged it out. One of them is a free turbine, so you have the PT6. And this is a GE Walter derivative, that they now call the GE Advanced Turboprop.
So the cold air comes in. It gets burned. And the hot exhaust gases spin this power turbine which has a shaft that drives the compressor. And it also goes back this way. There's a second. I think one of them drives the compressor, and there's a second wheel there that is exposed to the hot gases and it will drive the propeller. So there's kind of a fluid coupling there through the air.
Whereas the Garrett Honeywell product has a direct drive transmission that's more complicated but also a little bit more fuel-efficient. So those are the two big designs. You get a lot more reliability with a turboprop if you need power. It's hard to make a piston engine reliable if it generates a lot of horsepower. The disadvantage is nobody's really figured out how to make these things for less than half a million dollars new in a box.
And the most popular airframes for the turboprop for things that you might fly, the Beechcraft King Air which is twin-engine, and the Pilatus PC-12 which is a single. Turbojets, which-- well, the FAA calls them turbojets. They're really turbofans because of the bypass air. We'll see that in the next slide. These are-- one thing to remember about them is they're normally aspirated.
They actually do produce less and less power as you get up to higher altitudes which is important for taking off, you know, in Colorado on a hot day. You have much lower noise and vibration-- maybe not if you mount one of them right over your ear. That's a Cirrus jet which is kind of a cool simple personal jet. They actually have sort of taken over the market starting in model airplanes going all the way up to the Boeing's and the airbuses.
And the only gap where turbojets have not taken over is in your family airplane, right? Your four-seater, your six-seater, that's where nobody's figured out how to make a turbojet that really makes economic sense or even sense for the range. They tend not to be as efficient at these low-power settings. The smaller the turbojet, the less efficient it has been, unfortunately.
So you wouldn't get the kind of range that you'd get out of a Cirrus or a Cessna if you stuck a jet engine in there. These are more vulnerable. A bird will usually just get Cuisinarted by a propeller, but if it goes into the jet engine then it can destroy it. So the single-engine jet, well, some single-engine jets like fighters they should have an ejection seat. The Cirrus single-engine jet has a parachute for the whole aircraft to float you down.
All right, turbojet, again, this is not really on the exam but it's just good general knowledge. The fresh air, it comes in here. It gets compressed by the compressor. Once again, that's normal aspiration, and so it's just a fixed-compression ratio. It doesn't get more extreme like with the turbocharger in a piston engine at higher altitude. Notice this bypass air. It's being spun, and that blade in the front is really acting like a propeller.
Here is where the compressed air gets burned and drives these power turbines that drive the compressor and the fan in front. So it seems like a really simple design, but there's a tremendous amount of engineering in the blades. And if anything is fabricated just slightly wrong, you know, the whole thing comes apart and explodes. So that's one reason I think they're in the million-dollar range for a typical business jet engine.
All right, so let's go back to our world, which, unfortunately, is going to be the reciprocating piston engine kind of like in your car. The designs-- I don't know-- the fundamentalists may or may not have changed that much since the Wright brothers, but certainly, the designs that are in your modern Cessna or a Piper-- the basic geometry, the engine design-- is really all from the 1950s.
The good news is that with numerically-controlled machine tools and such, they're much more reliable than they used to be. Engine failure was an everyday event at a busy airport in the old days. And now, you know, I've flown for, I don't know, 4,000-plus hours with Pistons and have never had any kind of real engine problem. The horizontal opposition supposedly smooths things out. They may ask you about that on the test.
Like the turbojet, it's normally aspirated, so the performance degrades as you go to hot and high elevations where the air is thinner. We'll talk about that in the performance calculations. You directly drive the propeller. So if the engine's spinning at 2,500 RPM, the propeller's spinning at 2,500 RPM. There's no transmission. The cooling is the air, it's called air-cooled.
And the air flows over the cylinders, but there's also a lot of oil circulating which has a significant cooling function. The lowest cost and simplest airplanes have a carburetor rather than fuel injection. And you'll get 200 horsepower or less out of a-- well, I guess the latest Lycoming's can do to 215 out of a 4-cylinder. You can get a little bit fancier. A plane like a Cirrus or the latest Cessnas will have fuel injection.
Turbocharging sounds like the ideal thing for an aircraft. Why wouldn't you want that for a machine that goes through airs of all kinds of different densities? It seems like the ideal thing. Just make the density back to sea level at least all the time, which is called turbo-normalization.
The problem is that they share an oil system with the rest of the engine, so when the turbocharger breaks, it tends to cause all of the oil to leak out of the engine. And now you have a broken-- you know, what had been a perfectly good engine with a broken turbocharger becomes a real emergency.
So people's-- you know, except out west where you really need the performance because you are going up high a lot or you're starting from high and hot, the turbochargers tend to be unpopular, although it's an ideal engineering solution. The more cylinders also the less vibration, so if you have higher horsepower or if you just want it to be smooth. Like the original SR20s came with six-cylinder, 200-horsepower engines because they wanted it to be smoother.
Rotax is the most popular engine in the light sport and experimental world. That's an engine that's a little bit more like a car engine. It revs pretty high. It's got a water-cooling radiator with antifreeze and the transmission gears that prop down to a lower speed. All right, here's your basic engine. So up here in the cylinder head, you have your intake, an exhaust valve, and your spark plug. Down here, there's the crankcase and crankshaft.
Here's an exciting-- this is kind of an inline-four, but you get an idea of the power strokes. Everybody used to know this in the old days because they were excited about cars, so they had a lot of car-related knowledge, and it's basically the same idea. But now in the Uber age, we can't take that for granted. So you've got your intake stroke top left, and then the cylinder. The piston is pushed back up to compress the air-fuel mixture.
The spark plugs ignite. Notice how there's two of them here. That gives you some redundancy as well as more uniformity of combustion, which pushes the piston back down generating the power. And then finally, the piston rotates up and pushes the air out. I think this might be called the Otto cycle. I think a German guy invented this-- or actually, a French guy patented it first, but maybe Otto reduced it to practice first.
But anyway, it's a European invention from the late 1800s, and it really hasn't changed much-- four-stroke cycle. OK, cooling, as I said it's a combination of the air and the oil circulation. The FAA wants you to know that if you're getting the engine is going hot-- again, this is probably not going to happen to you here at level.
But if you're climbing in your little airplane out of Wyoming on a hot day, you might end up having to accept a slightly lower climb rate, boost your airspeed a little bit, and lower the nose to get more cooling air. The mixture control, you don't see this in cars because they have automatic systems to adjust the air-fuel mixture and get the right amount of fuel in there corresponding to the density of the air.
But in aviation, you know there's your little mixture knob where you can lean out the mixture. The fuel-air mixture, you start out rich when you're taking off because you're at a very high power setting, and the extra rich mixture cools the engine. If you go really extreme with this, like you just taxi around for half an hour with the mixture control at full rich, in some engines you may end up getting lead fouling on the spark plugs.
If you're up cruising and you lean it out too much then the engine's going to get a little bit rough, and some of the temperatures will go too high. On the modern aircraft, you have very sophisticated engine monitors that tell you all kinds of information about what the temperatures are. They may help you lean to the optimum mixture. If you have an autopilot, it's kind of a good way to invest some of your time once you're in the cruise.
Carburetors, how do they work? If you fly a Piper or a Cessna you can say that you have a jet-powered aircraft because there is a jet in the carburetor that's letting the low-pressure air and this venturi pull little bits of fuel vapor out into the-- well, I guess that throttle looks pretty well closed, but ideally the throttle would be kind of open, and the air-fuel mixture would be going into the cylinders.
Carb icing, so remember, lower pressure-- as the pressure falls the temperature will also fall. So even if it's not below freezing outside it can become below freezing in the middle of the carburetor, and, therefore, water vapor that has been in the air can turn into ice and eventually block the airflow to the engine. So they tell you, look, well, just detect that by loss of RPM. You know, you're flying along.
You should be watching the RPM gauge. So obviously, you would notice this and take the correct action, instead of having a machine that would figure this out and do it for you. I guess one reason they don't want to just have carb heat on all the time is that it robs the engine of some of its last 10 or 15 horsepower or whatever. So the carb heat because it makes the air hotter, it's just like going up to a higher altitude or flying on a hotter day.
The air is going to be thinner, and, therefore, there are fewer molecules of air to combust, and you won't get as much power. Question.
AUDIENCE: What generates the carb heat of the engine?
PHILIP GREENSPUN: What generates the carb heat? That's a good question. Same thing that generates the cabin heat. You basically run the air over the exhaust system before it goes to the carburetor or before it comes into the cabin. Good question. Yes, so I think 1950s cars had some kind of automatic mechanism for this-- it was, you know, buy a metal valve, and I don't know. The mixture control hasn't been seen in automobiles for quite a long time.
The ignition systems of aircraft is actually pretty well designed. You have magnetos. You can see them here and here. You can see him here at the bottom. The left and right magnetos are geared into the engine, and they generate their own voltage. So basically, you don't need to have a working battery or working alternator to have a running engine unlike in a car. All you need is these. One of the magnetos is still connected and working properly.
So it's totally separate from what you might think of as the aircraft's electrical system. And for further redundancy, each magneto is connected to half of the spark plugs such that if you lose a magneto, you still have one working spark plug in each cylinder. Does that make sense? You can see that in this drawing here where each cylinder is being fed either from the green or the blue magneto to one of the two spark plugs.
And you can test to see if it runs smoothly on either magneto. Your ignition switch on the airplane will have a right mag only switch, a left mag only switch, or both mags, which is where you have normal operation. But when you're testing on the ground you'll typically see if it works on either magneto. OK, abnormal combustion, what can you get? You can get detonation which is the mixture ignites before the spark ignites.
Or pre-ignition, which is the slow burn before the spark. And detonation I think is probably the one that's more common. And it could be caused by improper leaning. Aviation fuel, basically 100 low led which is dyed blue is all that you're going to see in the continental US. In the future though-- so low that means it's lower in less than the stuff that they were making in the 1930s or whatever. It's still pretty high in led.
It's like the super-- it's like the premium leaded gasoline, I think, of the 1970s. Most engines actually don't require this. It's just that it takes so long to get anything done in aviation that they can't put an alternative certify and get an alternative fuel to the airports. But, you know, your typical Cessna or your Robinson R44 with the carburetor, it doesn't need this lead that's in the fuel and then gets spewed out in the atmosphere. It's kind of a tragedy.
And it's been made worse by this ethanol stuff. So the government says you have to put ethanol in car gasoline. So you can't just go and take premium car gas from the gas station and pour it into your aircraft from a technical point of view-- even if it were legal regulatory-wise-- because the ethanol will destroy a lot of the fuel system in the aircraft which wasn't engineered for it. But if you did have ethanol-free-- there are a few places that have mogas.
They get special ethanol-free car gas, and lower-performance aircraft engines will run just fine on them. There have been committees-- you know 20 years is kind of like the standard unit of time in aviation. So for about 20 years, people have been working on some kind of certified unleaded gasoline that will work with most engines. If you see clear fuel in the sump, it usually means the airplane's been left out in the rain, and some water has gotten in there.
And you got to make sure that you drain that out. So there's drain plugs on the bottom of the wings typically, and maybe one near the bottom of the engine that you can see if any water has gotten into the fuel system. Jet fuel is hazardous to piston engines. The nozzles are designed so that they're physically incompatible. You shouldn't be able to put jet fuel into a piston engine. Occasionally it does happen.
I know a guy who was flying his Piper Malibu all around the world, and he got some barrels of fuel delivered to him, and he had to pump it himself. And I don't know, for whatever reason, there was jet fuel in there.
AUDIENCE: So Philip, you might want to kind of stop [INAUDIBLE].
PHILIP GREENSPUN: Is the pizza here?
AUDIENCE: No, not yet, but it's noon.
PHILIP GREENSPUN: Yeah, well, whenever the pizza is here. I think we're-- yeah, we'll take a break as soon as the pizza shows up.
All right, fixed-pitch propellers. So your most basic trainer has a regular propeller that its angle relative to the hub never changes. There's also in higher-performance aircraft, constant-speed propellers where there's an adjustment mechanism that you can read about-- a mechanical collection of springs and spinning weights that will try to keep the propeller at 1RPM, and that can be a lot more efficient in different phases of flight.
You can see from this that if you have a constant-speed propeller, the efficiency across a huge range of speeds is roughly constant, whereas if you have a climb prop or a cruise prop it's only working optimally at certain airspeeds. All right, so if you have a constant-speed propeller you're now going to have free power levers. That's the business end of it and from a pilot's point of view.
You'll have throttle which is the black one on the left, the blue prop-speed control, and then the red mixture. The FAA, they may ask you about this. You know they have this doctrine. It's not really true of all engines, but the idea is that you don't want like a high power setting and a really low RPM setting. They claim that that's making the propeller take such big bites of the air to keep the low speed that it's somehow stressing the engine.
But, again, not every engine manufacturer agrees with that. All right, let's talk about-- we'll start in on flight instruments until our pizza arrives. So this is going to be your reality I would say. Some of the test stuff concerns the steam gauges of the past that you still see at flight schools. And that there are some things that we can learn from this though that we'll get into. There are some advantages.
But anyway, let's start with this six-pack because actually, the electronic stuff is mostly trying to replicate what was done during World War II. There's very little in a modern aircraft that isn't some kind of upgraded version of what was in a Boeing B29 bomber, so it's worth kind of knowing where it came from. So you've got your six-pack. You've got your airspeed indicator. On the top left, you've got your attitude indicator or artificial horizon.
You've got your altimeter on the top right, vertical speed coming down around to the lower right, which tells you how fast you're going up or how fast you're going down, a directional gyro which is like a stabilized compass showing you where you're pointed assuming it's been adjusted properly. It has to be reset to the compass in flight every now and then. Ah-ha, is our pizza here?
AUDIENCE: I wish I had pizza.
PHILIP GREENSPUN: You didn't see any?
AUDIENCE: No.
PHILIP GREENSPUN: Oh, no. OK, and then you have a turn coordinator which is another backup gyro that tells you whether you're turning left or right and also the coordination. That's just a ball. We'll get into that in a little bit-- about whether the airplane is kind of pitched-- improperly yawed. All right, so it says electric. For a gyroscope to keep spinning that means it's being powered by electric.
What else could it be powered by? Does anybody know? What else is there besides electricity that can make this thing spin?
AUDIENCE: A vacuum.
PHILIP GREENSPUN: Yeah, a vacuum. So in the old days-- you know, again, this is not as common-- you'd have a vacuum pump on the engine that would generate a force to keep these gyros spinning. And those were often failing which had some pretty bad--
AUDIENCE: So these are the current reality of many of our planes?
PHILIP GREENSPUN: I don't know of how many-- yeah, I guess there's a few Piper Warriors. Yeah, that's a good reason not to take like the most basic airplane into the clouds. Because the idea is that you're supposed to sort of notice. Do they have-- I don't know. I guess some of them have a little warning light when the vacuum pump fails. But in the most classic situation, you're supposed to notice as the pilot in the clouds that this artificial horizon is failing and it's inconsistent with this instrument.
And then you decide which of the two to believe. Generally, it's this one because it's more viable. And then you continue your flight to the cloud without the artificial horizon. Anyway, that's ridiculous obviously. So, you know the real IFR airplanes these days have two attitude indicators, both powered by electric. And you don't have to sort of notice the subtle sign of them failing.
There's just a big flag that pops up when they fail-- you know, if they lose power for some reason, for example. All right, the pitot-static system. So how do you know anything about your airspeed? There's a tube somewhere on the airplane usually hanging out under the wing in your typical trainer or next to the nose. You see them on jets where the ram air comes in, and the more ram air pressure there is, then the faster you're going.
That can be compared to the static pressure which comes in from a static port usually on the side of the airplane. It has to be in some part of the airplane where the airflow is suitable for a static port. So the ram air feeds only the airspeed indicator, and the static source has to feed the altimeter and the vertical speed.
You've got to read this book. There's a couple of questions about what happens if the pitot tube gets clogged or if the static port is blocked and taped over, and that makes the instruments read inaccurately. So I'd read about that for the test. OK, airspeed indicator, here's what I was talking about how in some ways the old stuff is better. So, look, here's a good example of how you get more information out of this than out of the speed tape on the modern instrument.
This gives you all of the aircraft's performance basically. You don't really need to refer to the manual to see a lot of important information. Well, don't fly-- you know, the speed tape might only show you from here to here-- from like 120 knots up to 150 or something. But here you're seeing, OK, let's not fly faster than about 208 knots. Unless we have the flaps out, let's not fly slower than about 58 knots.
And even with the flaps hanging down, we don't want to fly slower than 55 knots because that's going to be where it's going to stall at certain CG configurations and at gross weight. OK, the yellow arc. Well, the yellow arc is to be avoided unless the air is smooth. So that was actually a great user interface.
And because cool jets have the speed tape when they make the glass panels for little airplanes which really don't need that kind of precision and speed control, they said, well, let's just have this thing that the jet pilots have. All right, how does the airspeed indicator work? There's brass in there, so it's a cool old-school Sherlock Holmes-style brass instrument. The ram air is coming in.
There's a diaphragm that moves some levers, and eventually the needle swings. Again, you know, you don't have to design one for your test. You just have to know that it's going to respond to the ram air. Some of these little symbols are worth knowing. Probably Va is the most important because it's on the test, I believe, as something that is not indicated on the airspeed indicator. That's the maneuvering speed.
That's the speed at which you can do extreme control inputs and not bend the airplane. Any faster than maneuvering speed it's possible that you could yank the yoke back so hard that you'd bend the wings a little bit or overstress them. The reason it's not indicated on the airspeed indicator is it varies by weight.
And it's actually higher-- that's something worth reading about-- it's higher if the airplane is heavier. It's one of the few speeds where weight is helpful because if it's really heavy, it's just harder to get the airplane to perform in some extreme manner. VSO is also worth looking at. That's basically the speed that's going to determine how fast you land. That's the speed that the airplane will stall on landing configuration.
So you usually want to be going at least 20% faster than that to give yourself a safety margin. OK, here's something from the New England Air Museum in Bradley, Connecticut-- another great destination about half an hour's flight away, huge airport, great FBO. They will give you a free car to use while you're there. It's called Signature Flight Support there. Anyway, so this aircraft-- I think it's a P40 from World War II, and it can go up to 500 miles an hour.
All right, altimeter, this aircraft is at about 10,000 feet. So notice it has this little triangular needle at 1 that's telling you that it's at 10,000 feet. And then the longer needle is reading in hundreds, so we're at about 10,200. And sure enough, we can see this is indicating you know 10,180, so 3 needles. Usually, the ones you're going to deal with are this one at the top here for thousands of feet and this one for hundreds of feet.
OK, so back in the old days before we decided to dig up all the coal and the oil and set it on fire, this was our standard atmosphere, and it was wonderful. And it was always 15 degrees at sea level. And it was always 29 or niner two for pressure, but now we've ruined it. The standard atmosphere is the basis for a huge number of calculations.
The altimeter, we're going to look at this again. It has these android wafers that, I guess, provide a reference pressure. And somehow the difference between what's in there and what's coming in will make the needles move. That's about as much as I know not being a mechanical engineer. An important factor though with all these altimeters is you can set it to correct it for whatever the prevailing pressure is locally.
So that's what you really need to know as a pilot-- that if they tell you the altimeter setting is 3053, that you turn it until you see 3053 in the window. It's worth knowing these altitude definitions. The most important for determining aircraft performance is going to be density altitude. That kind of tracks the actual number of molecules that are in a given volume of air. If you're flying along, you'll be looking at indicated altitude. That's kind of how aircraft tend to be separated.
Everything else is kind of nice to know. Pressure altitude, we talk about it a lot, but I don't think it's really often used in practice for much. Actually above it-- I shouldn't say that because above 18,000 feet it's used. You always set the altimeter to flight level-- you know, 220 instead of 22,000 feet.
If the temperature standard pressure altitude and density altitude are going to be the same-- so that would be, again, 15 degrees at sea level and cooler as you go up-- the pressure altitude will also equal the true altitude. So that's the actual height above sea level, again, in standard atmospheric conditions. We'll talk about this at a few times in this class.
But when it's hot the entire Earth's atmosphere expands, and if we were to cool the earth down near absolute zero, the atmosphere would contract. We might read on our altimeter that we were at 18,000 feet when we were only, you know, an inch above the ground because the Earth's atmosphere had shrunk to only two inches in size.
All right, when you're flying indicated altitude there's an expression called "high to low, look out below." Because if you go from an area where there's a high-pressure setting to an area where there's a low-pressure setting, you'll inadvertently descend by 1,000 feet if you just follow what the altimeter is telling you without resetting the altimeter. Same issue when you go from high temperature to low temperature.
So I just came back from Jacksonville, Florida, in the Cirrus, so I went from high temperature. And at an indicated altitude of 3,500 feet, I was actually a bit higher above the earth than here in cold New England. So that's another thing to be aware of, and this becomes important for instrument flying in very cold conditions like up in the Canadian Arctic.
If you fly indicated altitudes when it's extremely cold, you'll be closer to the ground than they budgeted for when they designed the procedure. OK, vertical speed, if you want to maintain 500 feet per minute climb rate or descent rate for your passenger's comfort, then this instrument is your friend. It does lag so you can't really use this to-- all these instruments are outputs, not inputs. Your inputs as a pilot are the attitude and the power. So you set a power.
You set an attitude like a little bit three degrees nose up, and then you use these instruments to verify that you're getting the performance that you want. Especially true for the vertical-speed indicator because the instrument itself has lag in addition to the lags that come from just the inertia of the aircraft. OK, under the hood it's gotten this calibrated leak. I don't really-- anyway, it's another fancy instrument than a mechanical engineer should tell you about.
All right, gyroscopes, the main property is you can use them for an aircraft instrument because it will try to stay where it is relative to space. It has to be corrected. In the instruments, it's really where gyroscopic precession has to be considered because think about it, you're going to be showing the pilot various things on the face of the instruments. So you want to sure that the deflections correspond to what's happening out there in the real world.
Again, this is covered in the Pilot's Handbook. This just tells you, again, if you kick a gyro up at the top, instead of the axis of the gyroscope rotating downward, it's actually going to yaw to the left. That's gyroscopic precession. Turn coordinator, it has a gyro to tell you that little airplane, if you're doing a standard rate turn, it'll go to this point here. That's a two-minute turn that'll take you two minutes to go 360 degrees.
And it's a backup to a vacuum-powered attitude indicator. It also has this completely separate function of a little ball in-- I think, it's in kerosene actually-- so it's a little ball in fluid. And in a perfectly coordinated turn, you should actually feel like you're just standing on the ground at 1G, and the ball should stay right there in the center. All right, turn coordinator, it can tell you whether you're slipping, skidding, or coordinated.
So if you're coordinated, as I said, it's just like sitting in a chair and not moving. If you're slipping, if you have any trouble visualizing this you can just reflect on the fact that you have to step on the ball to fix the problem. So here your airplane's in a right turn, and it's telling you you have to step on the right rudder and bring the nose more around to the right in order to be coordinated. So that is a slipping turn. So here's our airplane.
We've banked the wings, but we've got the nose still kind of pointed where we used to be going. So it's telling us you need to kick a little bit of right rudder and have a coordinated turn. Skidding is the other way. For some reason, we have too much right rudder in there, and we need to back off of it or hit some left rudder and bring it back. Again, this is something, it'll become-- you know, eventually you develop a sort of a feel for it in the seat of your pants, and you'll just do it naturally.
And actually, modern aircraft don't have a whole lot of, what's called, adverse yaw, so if you try to turn them, they're not really going to get out of coordination to any large extent. All right, attitude indicator. If you're either in level flight or on the ramp, this little airplane pointer here in the middle-- that little orange thing-- is just something that you can rotate up and down. It's basically just a reminder for you of what attitude will produce level flight.
It might be a couple degrees pitched up or down, but you just set it to whatever it is. This is a gyro that's free to move in two directions, and as it moves, it moves this bank index, I guess, on the face of the gyro is what they're calling it-- or maybe that just is the bank index. It's moving the whole artificial horizon on the face of the gyro.
So the important thing is it's a gyro at 2 gimbals. In aerobatic airplanes, this design doesn't work very well. It tends to get damaged by all of the extreme maneuvers and g-forces. As a pilot, what do you need to know about this? It's worth knowing how to read it. In your flight training, you'll do, so-called, steep turns where you'll bank to a 45-degree angle.
It's mostly a visual maneuver where you look outside, but the attitude indicator is a good reference, again, to see whether the picture that you've got of a natural horizon actually does correspond to a 45-degree bank. If you go up, I think if you go beyond 60-degree banks, it's time for your aerobatic airplane and your parachute. That pointer at the top generally will be pointed up at the sky however you're maneuvering. We'll see this in a moment here.
So you can see, let's just pick a level right turn. So over here, you say, well, that thing at the top isn't really pointed up at the sky. It's just pointed off to the left but think about it. They're just showing you the instrument as you would see it in the cockpit. So because the airplanes turn to the right, that top pointer would actually correspond to straight up into the sky.
But as you see, it's kind of the same picture you would get. You're more or less not pitched but you are banked. If you're going up climbing right bank, you see you're just pitched up a couple degrees. There's a little bit more blue here versus here. As an instrument pilot, you'll get very good at seeing small differences-- just one or two degrees of bank or pitch here.
We're about to go into the magnetic compass, so let's take a break here and we'll wrap this up after everybody has their pizza. A few things to remember about magnetic compasses, and then we're off to Oshkosh which is going to be more fun. The most important thing is that it only works when you're straight and level. And the fundamental insight for this is the compass is a horizontal thing. It's only designed to move horizontally.
So if you start tilting it, tilting your aircraft, one big part of it-- big part of the problem is the compass tries to still point itself towards the magnetic north pole which will cause it to rotate a little bit. It's a little bit hard to explain without thinking about it and reading about it in the Pilot's Handbook. But the takeaway is that because it has inertia, and because it's not really fully free to rotate to point in three dimensions down to the north pole, you get all these bizarre errors.
Like if you turn away from a heading of north, it'll initially show a turn in the opposite direction. And if you accelerate because of the inertia, it'll-- let's see, accelerate north, and yes. All right, we'll get into that in a moment. Even I can't remember it. Just remember don't look at it unless you're straight and level. The true magnetic-- I think actually the north pole-- the magnetic north pole, I heard recently there was some kind of significant movement.
Anyway, it's moved quite a bit since 1632 as you see here on this chart. Because true north is not equal to magnetic north, and the kind of primary source of direction that you have in your aircraft is a magnetic source, on the charts, you end up getting these isogonic lines that tell you how much correction to apply. So here you can see 14 degrees west.
That tells you-- because if east is least, and west is best-- if we want to fly straight true north, we actually have to steer zero plus 14. So zero is the true north course, add 14. And if we see 14 on our compass, or between 10 and 20 on our compass, between 1 and 2 I guess it would be indicated, then we're heading true north. If you forget all of this stuff that I'm telling you-- which I hope you will because this is a very tedious area-- you can cheat.
So look at this VOR. The VOR tells you this is the Block Island VOR. This is another great place to fly. So you land there. You walk to the beach. You skip out on the ferry ride. This is the best arguments for general aviation aircraft are almost always places that are islands, and you can't drive there on your Honda Accord even if you wanted to. So but you see here, the zero magnetic line is not aligned. The charge is in true north. So there is your true north.
There is your zero magnetic. So you can just see that the variation here is about 14 degrees. You wouldn't even need-- and you can also that east is least, west is best rule, you can rederive it just by looking at any VOR. Except don't look at a VOR in Florida because that's where there is no variation. But almost any other VOR you can look at and see what the new rule is.
All right, magnetic deviation. If you have stuff in the airplane, it will cause the compass to be a little bit inaccurate. So they give you this compass card here underneath, which will give you usually one or two-degree changes at the most for how you would-- you know, if you want to fly north magnetic heading, you might actually steer, I don't know, zero-point-- steer a course of one or two degrees.
You know, if you bring stuff into the aircraft that's heavy and metal or magnetic, that can affect your compass. And actually, in an aircraft with heated windshields, if you turn on the heated windshield for anti-icing, and in jets, it's kind of standard to fly with them on all the time, the compass becomes useless. The heated windshields throw it off by 90 degrees. All right, in the lowest end airplane, they'll have a directional gyro.
Because of all of those compass errors, you as a pilot, will basically, just every five or 10 minutes if it's a really old and bad DG, or maybe every half hour if it's a decent new one, you'll just look up at the compass when you're in level unaccelerated flight, and you'll reset with this knob here down at the bottom. You'll twist that to align the directional gyro to the compass. So the compass is the authority, but it's only an authority when you're straight and level.
OK, under the hood, notice that this, unlike the attitude indicator, this only has one gimbal. And that's really the takeaway for why the attitude indicators tend to break more often than these other instruments because they have two gimbals and they're just a little more fragile overall. Horizontal situation, everything in flying, they've tried to make it more idiot-proof, and the HSI is a great example of something that was figured out, I don't know, maybe in the 50s or maybe earlier.
They said, what if we just take that DG and we put an indicator on here. This is showing we're trying to go on a heading of about 010-- just slightly north. That's this yellow pointer. We are actually going on a heading of about 020. Maybe that's because of a wind correction. There's a wind coming from the right. And it also integrates your line. See it shows you with this course deviation indicator that you're slightly to the right of the course.
The course is a little bit to your left. And actually, this person, I think, this pilot is flying an Instrument-Landing-System approach. This yellow trapezoid there on the left and right is showing you where you are relative to the glideslope. So you're right on the glideslope. So basically, all the information that you need for your flight other than the attitude is on this single instrument.
If you have everything set up correctly the radios or the GPS, you really don't need to look anywhere but here and at the attitude indicator. It's a pretty nice idea. And this is replicated in all of the glass cockpits. All right, technically advanced aircraft, here's a photograph of the latest and greatest Cirrus SR22 or 20 cockpit. You have two big screens.
One's usually called the PFD-- primary flight display-- one's the MFD-- the multifunction display-- that shows you maps and weather and traffic. This idea came from the big jets from the 80s, I guess, and 90s. It was adopted in the Cirrus in 2003, pioneered with an MIT spin called Avidyne which is a local company. Garmin kind of took things over.
And most of the aircraft today come with Garmin. G1000 is a brand name that you might have heard for a Garmin product. It's pretty popular. In some ways, people with older aircraft are almost better off right now. There's this huge stream of retrofit stuff that's very innovative, more so than the comprehensive glass panels. And usually, for $30,000 or so an older plane can be brought up to the modern standard. OK, what if things are not going well at all?
The ultralights tend to come apart in flight. Certified airplanes virtually never come apart. They're just so over-engineered that wings don't fall off, struts don't fail. But the two brothers-- the Klapmeier brothers, from Wisconsin, I believe-- although the company they started, Cirrus, is in Duluth, Minnesota-- they say, look, this parachute would give people a lot of comfort, and there are some situations in which it could be useful even in a certified airplane. So they added it in the Cirrus.
And you pull it, and the rocket chutes the parachute out, and you float down. It's becoming more and more popular. I thought it was a payload-hogging waste of money when I bought the Cirrus in 2005. And now that the engine has 2,000 hours on it, and I'm flying at night, I think that's pretty good. That's a pretty good parachute. I don't even mind the $15,000 that we paid to have it all repacked at the 10-year point.
All right, summary, so piston engines, they're very fuel-efficient compared to jets at these power settings. They have quirks which sounds bad. You know, one cylinder might be running rough, but it's better than in a jet world where if things aren't perfect, the engine just quits or explodes or something. So turbine engines seldom go bad, but when they do go bad it's often with no warning.
Here's your six-pack. The altimeter, remember, is essentially measuring a percentage of the atmosphere that you're above or below. Compass is full of errors except when you're straight and level. And also the aircraft manufacturers are really systems integrators. They're buying the engine from somebody. They're buying these instruments like the Garmin G100 from somebody. Study pitot-static failures and the compass areas. And they're on the exam, and they're not intuitive.
Questions. This is another picture from that air museum in Bradley. They have one of the handful-- it's not flying, I don't think, but it's restored-- a Boeing B29 bomber. And if you want to know why I get defriended all the time on Facebook, I posted this picture of their turret computer, and I said I found the original iPad in the museum. That was hashtag not funny.
[LAUGHTER]
All right, any questions or should we go into Oshkosh? We can have questions. Don't ask about those brass and neural things because I was a math undergrad and EECS grad student.